Spectral Measurements of “Neon” Lights in Artworks for the Identification of Gas Composition and Color Characterization
Measuring gas-discharge tube with a handheld spectrophotometer
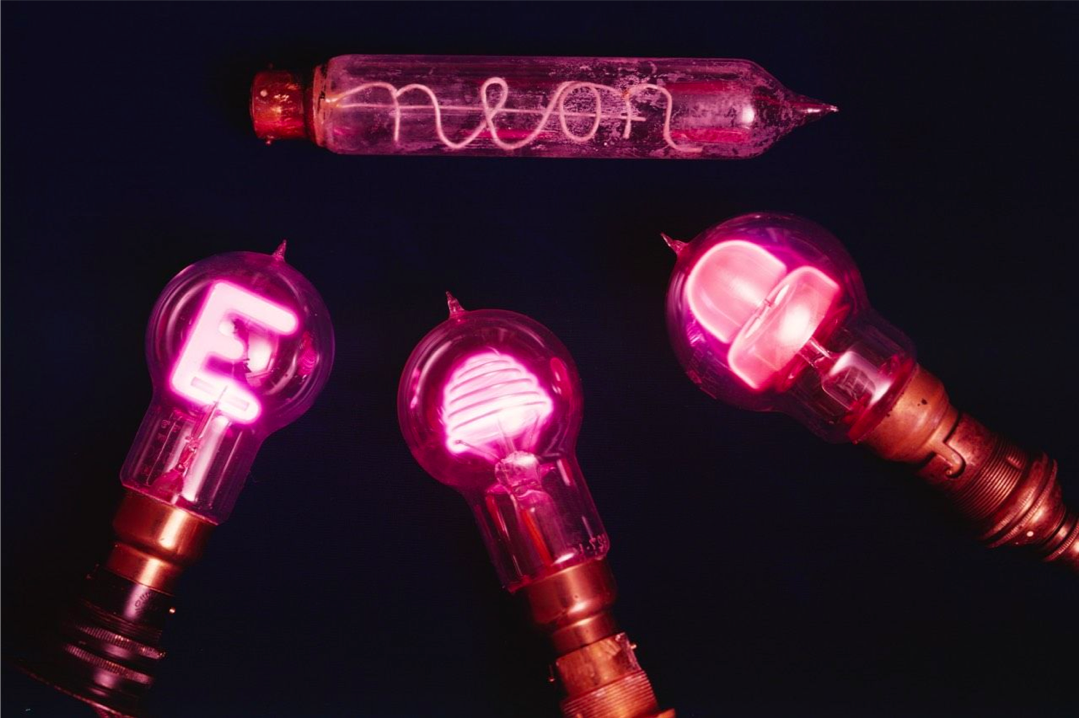
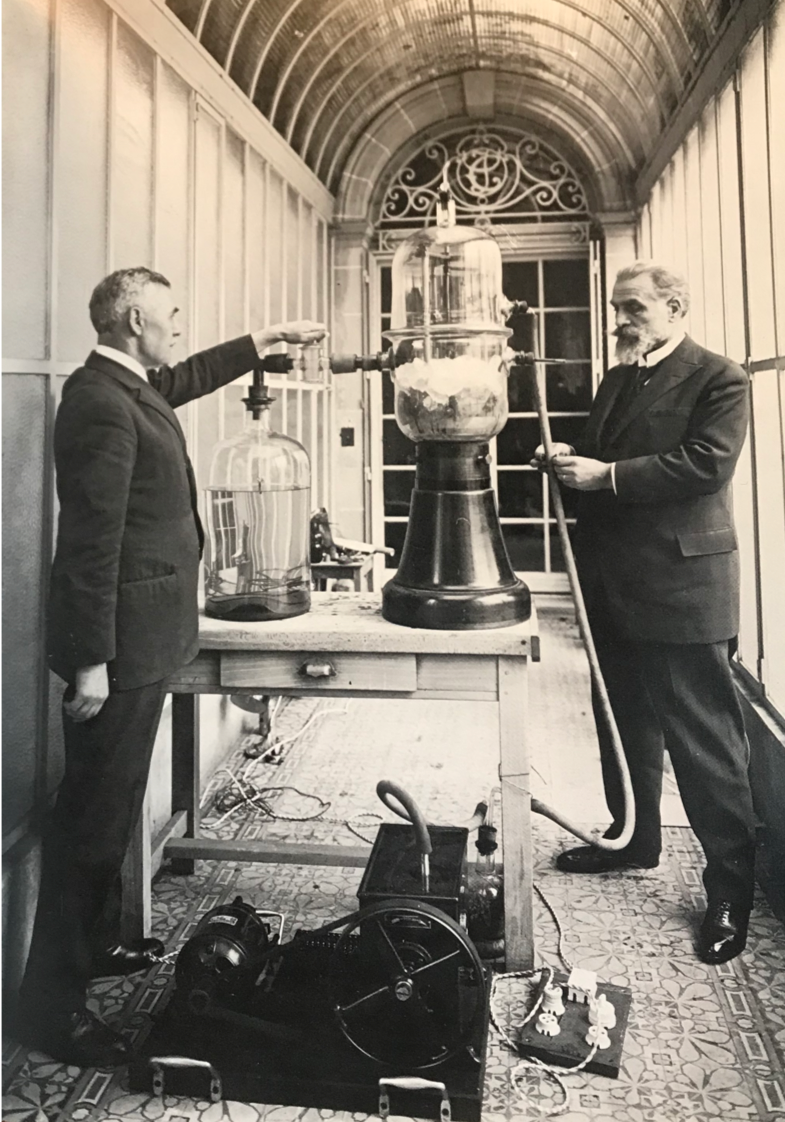
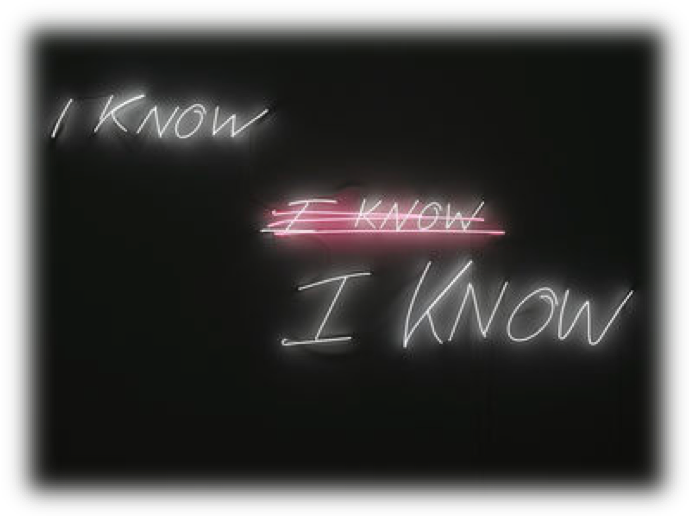
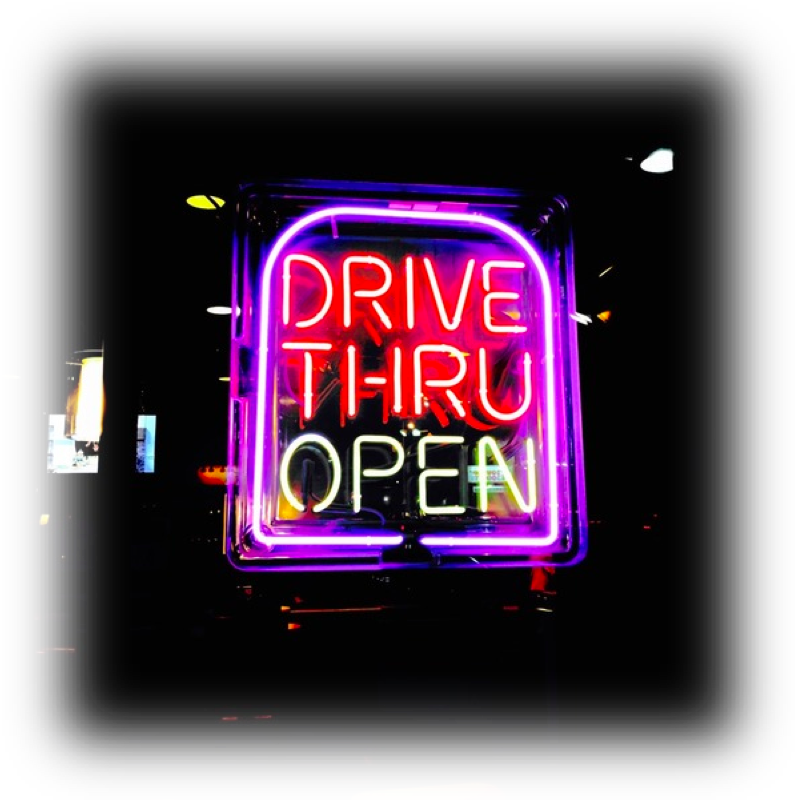
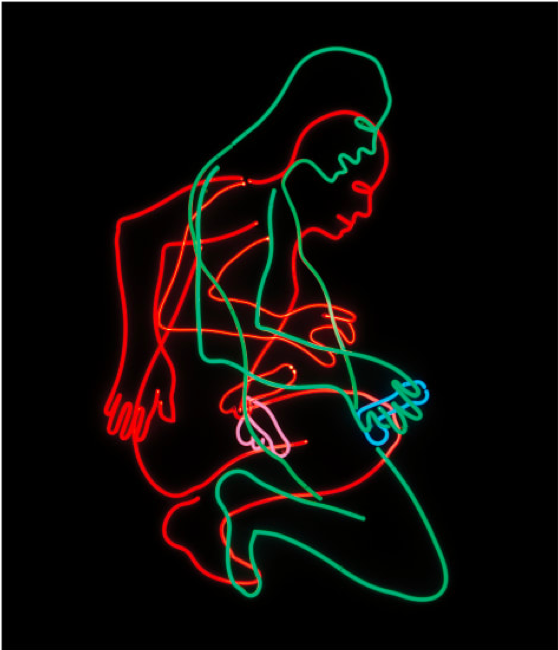
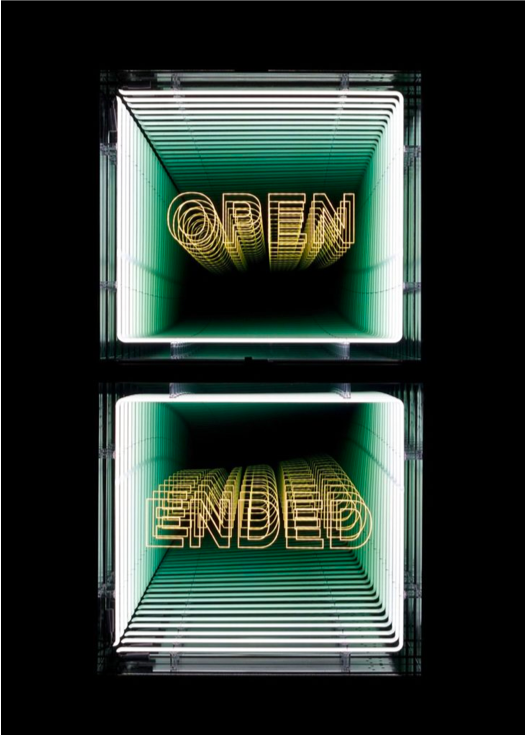
Gas-discharge tubes—more commonly known as “neon” lights—have been used in signage since the early 20th century in artworks soon after. Modern and contemporary artists including Joseph Kosuth, Tracy Emin, Bruce Nauman, and Lucio Fontana valued this medium for the variety of colors of light the tubes can produce as a result of different combinations of noble gases and glass tubing. These artists often relied on trained fabricators to create their designs, restore, and replace components over the lifetime of the work. Aging units and their electronics present condition issues such as color shifting, sputtering, and failure. Currently, it is a common and accepted conservation practice to replace both the tubing units and electronics as needed, but documentation of the intended appearance of light or material composition of the gas-discharge tubes is rarely accessible. This makes it difficult for collections care professionals to identify original materials and subsequent condition issues which creates a dependency on fabricators and restorers. They depend on their extensive training and knowledge of workshop practices to replicate these components. However, it has been observed that replicated components are not visually faithful to the original intended aesthetic qualities sanctioned by the artist. The need for identification of gas composition is especially crucial for gas-discharge tubes in artworks that require replication has been recognized in order to faithfully reproduce the aesthetic qualities of the tubes, the composition of the original materials.
This research addresses an experimental technique for the identification of gas-composition of gas-discharge tube units used in works of art and cultural heritage. It involves use of a handheld spectrometer to measure spectral power distribution of the emitted light and match the characteristic peaks to that of known noble gases. In addition to the identification of the gas-fill, the spectrometer can measure chromaticity, which locates the color of light within an international color-space standard, CIELAB 1976. Together, this data can be used to faithfully document the color of light produced and identify gas compositions of newly fabricated and aged artworks, which can aid in the identification of condition issues and assist fabricators for future replacements. An experiment at the end of this paper describes a workflow for measuring the spectral power distributions and chromaticity of a number of different gas-filled tubes used in a newly fabricated artwork.
This research was presented at 2021 AIC/SPNHC Joint Virtual Meeting during the Contemporary Art Network (CAN) session.
Introduction to “Neon” Technology
Diagram demonstrating how cold-cathode gas-discharge tubes produce light. Diagram: Lessons In Electric Circuits copyright 2000-2017 Tony R. Kuphaldt
This technology is integral the understanding of my research goals so I will begin with the most brief introduction. Neon light technology as we know it today was introduced to the public by Georges Claude in France in 1910 (patented in 1915). Claude actually built upon a phenomena discovered in the 1890s that a glass tube filled with neon gas and a drop of mercury would glow by shaking it in the dark.
Not long after, this technology was used to create architectural elements, advertising signage— and since the 1950s, artworks designed by fine artists.
Objects that colloquially fall under the category of “neon”—whether artwork, architectural/advertising element, or light sources—should technically be referred to as cold-cathode gas-discharge tubes. Unlike a hot-cathode, which is electrically heated by a filament, cold-cathode lamp technology depends on a positive and negative cathode exciting gas molecules to emit light.
The color of light produced by gas-discharge tubes is controlled by the following parameters:
Nobel gas type (aka gas fill)
Gas fill pressure
Glass tube composition
Glass tube diameter
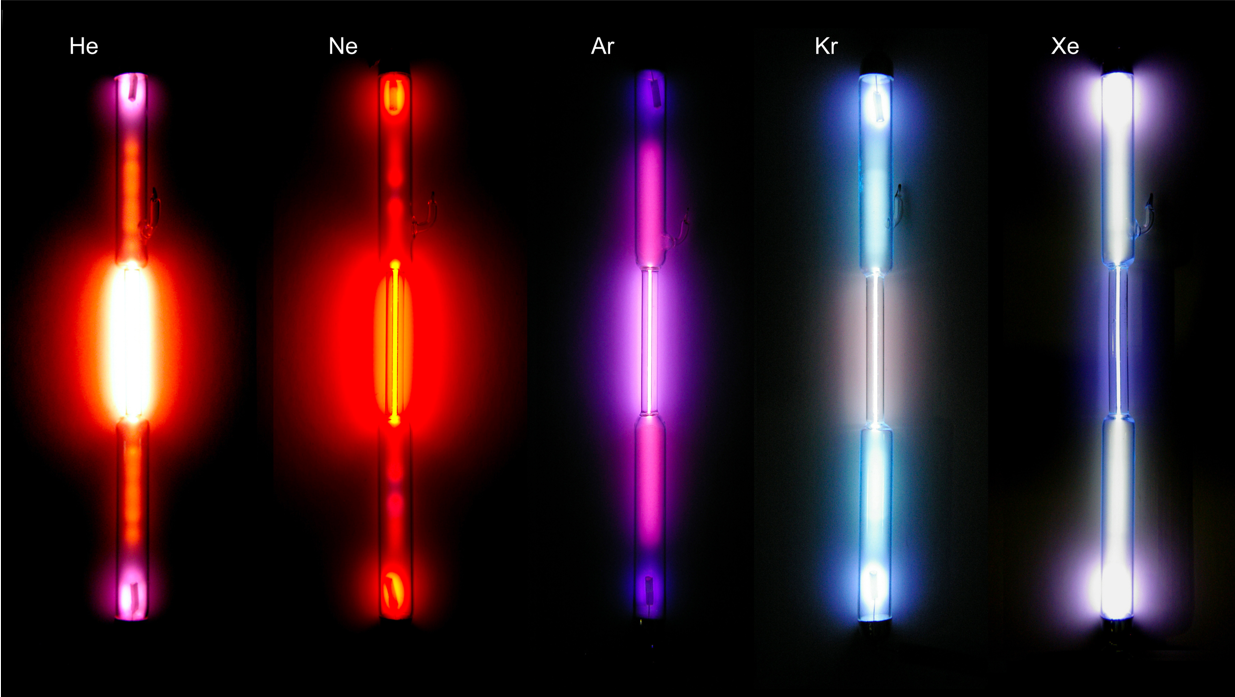
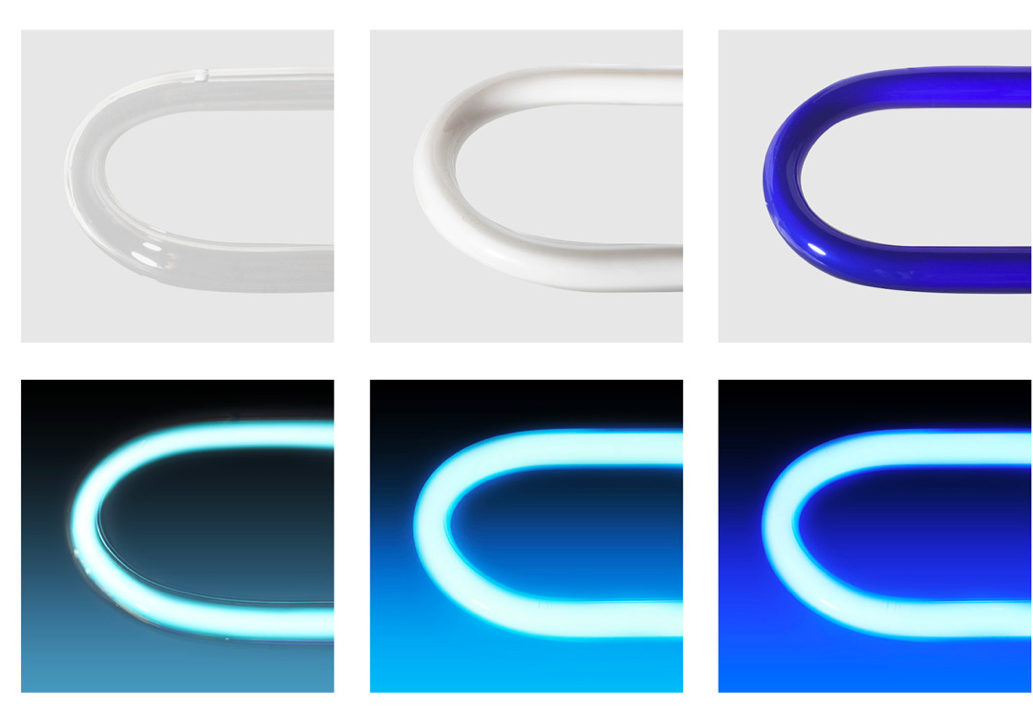
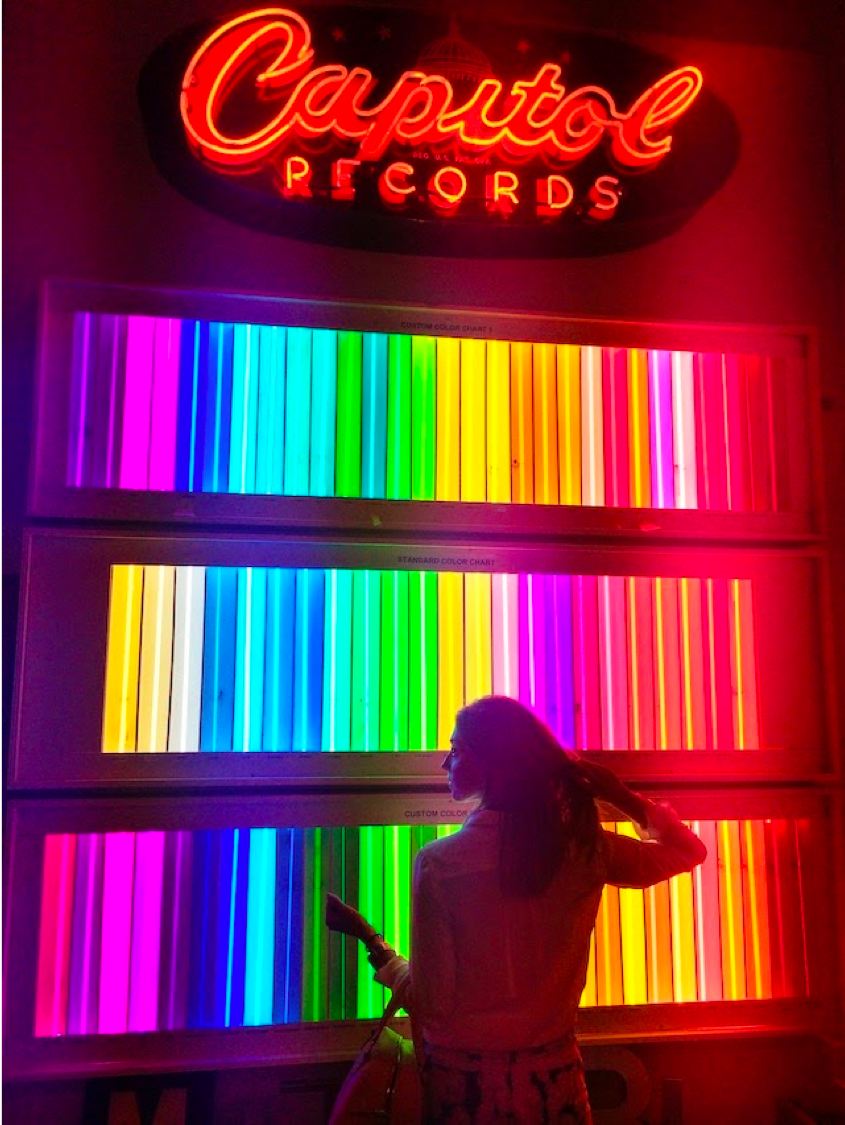
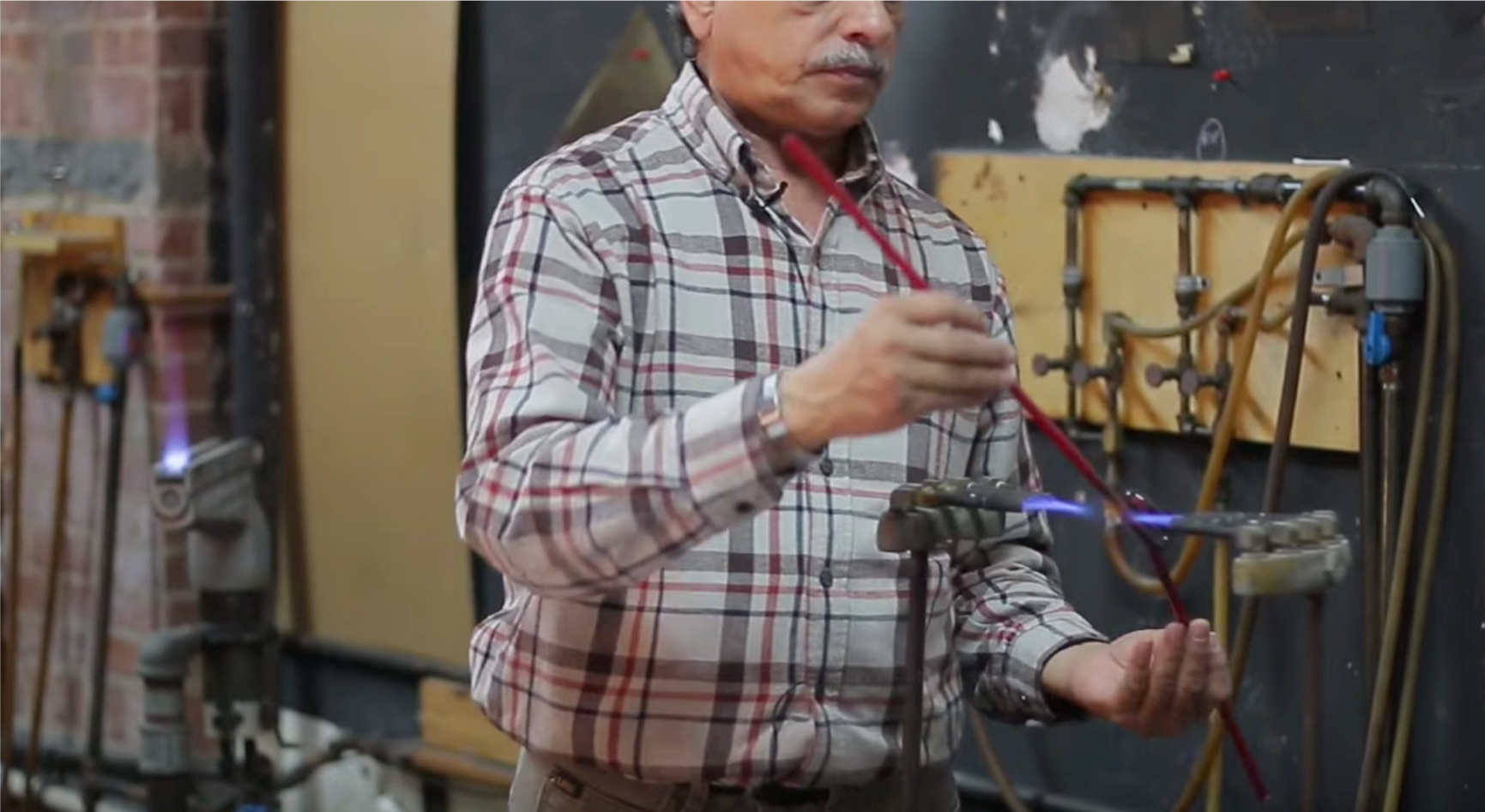
Gas fill and glass type are the most important materials to identify on gas-discharge tube artworks because they significantly affect the visual properties. The composition of the glass (ex. borosilicate, soda-lime, etc.) is as not crucial to document as the type (ex. clear, colored, phosphor-coated). Glass type can be distinguished easily through visual examination when the gas-discharge tube unit is powered off. The clear tubes are colorless and transparent, while the phosphor-coated tubes will exhibit white or pale colors coating the interior of a clear tube. The colored tubes are saturated, bold colors that are achieved through the addition of metal-oxides into the glass before it is shaped into the tube shape. It is not possible to accurately identify the composition of the gas-fills through visual observation alone, even when the light is turned on. Additionally, the names of the tubes are rarely related to the type of the tube or gas fill and are not standardized between fabrication shops. Fabricators can make educated guesses based on the perceived color and tube names, but as was seen in an Guggenheim example below, their predictions can be inaccurate.
The glass tubes are bent into shape then filled with a single noble gas or combination of gases: helium, neon, argon, krypton, and xenon. Each of these gases alone produces a different and characteristic color distinct spectral power outputs. Many gas-fills also include 100-1000mg of mercury which vaporizes and intensifies the light level and color—especially in phosphor coated tubes to produce the proper fluorescence. Electrodes are spliced to each end of the tubes, and are connected to transformers, allowing for the units to function.
The complete units include glass tubes, enclosed gas, transformers, electrodes, tube supports, and sometimes paint coating to black-out sections that are not intended to be illuminated. They are composite objects, and thus present various conservation issues such as sputtering of the light, development of dim or dark sections, and total loss of function. Depending on the tube’s manufacturing, frequency of use, and exposure to extreme environmental conditions, gas-discharge tube units’ lifetimes can range from 10,000 to 25,000 hours. For art and cultural heritage collections these condition issues warrant conservation/restoration or total replication. While fabricators have an intimate knowledge of the variables that control the color of gas-discharge tube systems. However, techniques are proprietary and are typically not shared outside of studios. The following experiment offers techniques to identify the materials used in these units that can provide valuable documentation for conservators and fabricators.
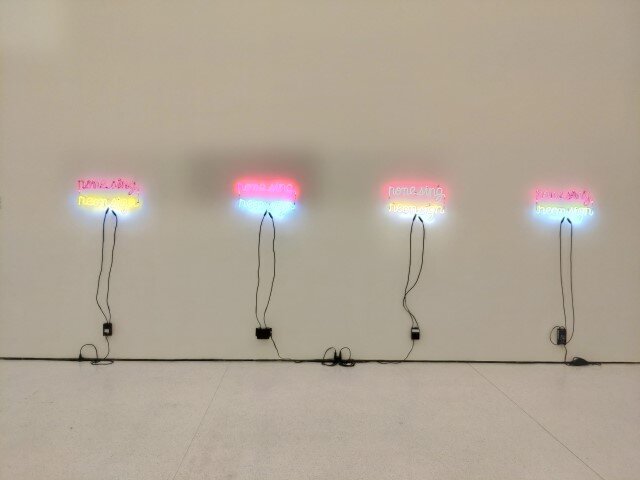
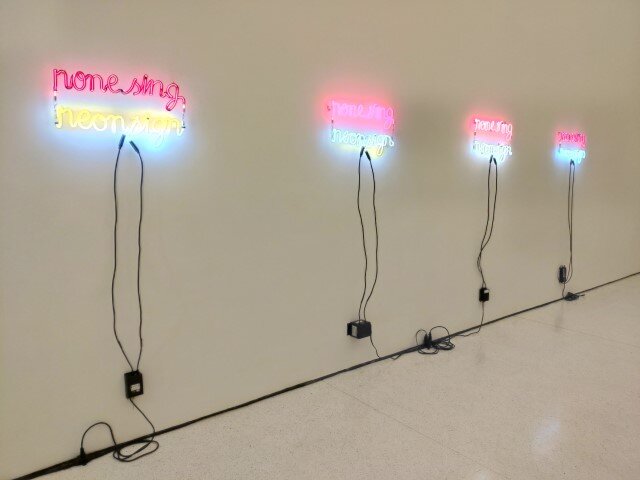
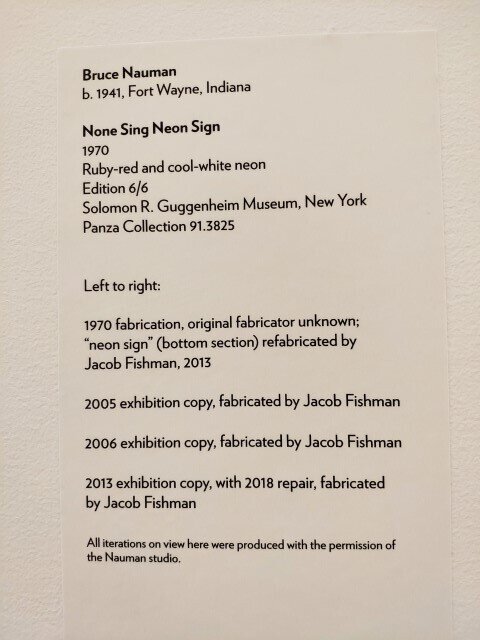
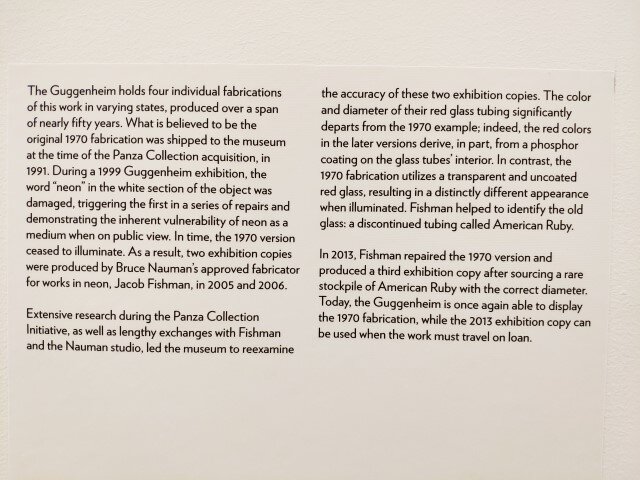
Case Study: Guggenheim
The most recent exhibition cleverly presented the restored original alongside subsequent exhibition copies to demonstrate the relation between colored light and gas discharge tube components. You can see from this photo that each unit produced significantly different colors and intensities of light. Through extensive research conducted by the Guggenheim and fabricator, the original 1970 materials were identified and a materially-faithful exhibition copy was created in 2013 seen on the far right. It is important to note that the restored original would have looked more like the 2013 version when it was originally created, but has aged and shifted color over time. This venture was explained only on the wall label, and perhaps warrants a full publication. However, as mentioned above, it is possible that the involvement of conservation was much less than that of the fabricators.
This example demonstrates that fabricators rely heavily on experience and intuition to identify the parameters that affect the color of the gas discharge tubes, and often the color appearance is not authentic to the original visual experience.
Identification of Gas Fills
Visual Identification
Like many material identification techniques, there are practical ways and highly technical ways. You can do some reliable gas identification by looking at the color of the ionized gas at the ends of the tubes nearest the electrodes if they are accessible. Most gas fills are either neon, pure argon, or argon mercury. The clear glass around the electrodes exposes the characteristic reddish orange of neon gas, cool bright blue from an argon mercury fill, and a dim pinkish purple produced by pure argon.
However, visual identification can be unreliable when more than one gas is used in a single unit, and when uncommon gas pressures are used.
Spectral Power Distribution
So to be sure of what gas fills you have, I developed this technique using a handheld spectrometer to visualize the light in a way that reliably distinguishes gas fills. Spectrometers produce spectral power distributions by measuring the energy levels at each wavelength in the visual light spectrum. Spectral power distributions are a kind of “fingerprint” of a light source, as they are the key to how all light sources render colors. Spectral power distribution curves describe relative energy emissions of a light source at each wavelength of the visual region of the electromagnetic spectrum (ranging from approximately 380nm to 780nm). Spectral peaks have been used to detect the presence of certain elements of materials for over two-hundred years.
Reference spectra of noble gas fills were captured by the spectrophotometer and can be compared to experimental spectra to identify their gas fills.
Case study: A Tale of Two Ruby reds
This technique becomes incredibly valuable for identifying tubes used in artworks or historical signs for documentation and preservation purposes. Certain recipes are chosen to achieve exact colors, and each come have their own aging properties. For example, ‘ruby red neon’ and ‘ruby red argon’ are produced by different recipes but are virtually indistinguishable. The differences become evident when we look at the spectral power distributions. We see distinct spectral peaks produced by each tube demonstrating that there are two different gas fills. If we compare these spectra to the noble gas references I created we see the distinct pure argon distribution produced by the top tube and the distinct neon spectral distribution on the bottom. So if one of these tubes were in an artwork or a sign that required replacement in the future, we are able to capture spectral power distributions and identify the gas fill before it fails
Fabricators can fill ‘ruby red’ glass tubes with either pure argon or neon gas depending on the desired environment or aging properties. The appearance of ruby red argon and ruby red neon is nearly indistinguishable. To distinguish the gas fills, spectra from each unit can be captured (left) and compared to reference spectra (right). We can see that the top left spectra most resembles that of pure argon, and the bottom left spectra most resembles that of neon.
Spectral Peak-Identification Script
The ruby red example is just a single comparison that I was able to make by studying the spectral measurements of the experimental units. There was so much data to parse through with the other 60 experimental units so I decided to spend my time making a python script that was scalable and sharable. I created a python script that identified the peak wavelengths from each experimental unit’s spectra and exported it to a master spread sheet. These values can be compared to reference peak wavelengths and gas fills can be identified by comparing numbers instead of spectral distributions. See this page for details on how I created and executed this tool.
Dozens of experimental gas-discharge units produced endless data to parse through.
Documenting Color
In addition to identifying gas fills, the spectrophotometer can also assist in quantifying the color of light by measuring its chromaticity. Chromaticity is the objective specification of the quality of a color regardless of its luminance and it is measured as coordinates in a standardized color space. This is actually how the Department of Transportation and National Bureau of Standards control the color of traffic lights. Photography fails in capturing accurate documentation of a colored light which creates problems for conservators and collections management professionals.
case Study: Documenting Color-Shifting
We can use chromaticity measurements to document the color of light at any point in a unit’s lifetime and compare the measurements to identify color-shifting. Color-shifting is a phenomena that fabricators have observed as either a result of materials’ aging properties or fabrication flaws. Long time employees of Brooklyn Glass have noticed that the color of this entrance sign has shifted, so I decided to compare the chromaticity values between the aged sign and the new experimental unit representing the same recipe.
The Brooklyn Glass sign was fabricated in ‘blue neon’— a cobalt blue colored tube filled with neon gas. When powered off, the sign is blue. When powered on, the sign glows reddish pink. Employees have noticed that the sign has shifted to a deeper red and lost its pink qualities. Additionally, the glass tube of one of the letters had been overheated which resulted in what is known as a ‘hotspot’ or a localized difference in color.
Let's ignore the color of these images and instead use chromaticity to document the colors. We can actually quantify the sign's color shifting with the captured coordinates on the chromaticity diagram. We can see that the color from the sign is a more red than the new sample which is reddish pink. This is valuable information to have now, as the sign will continue to shift and we can take more measurements in the future and actually plot the colorshifting which has never been done!
In addition to documenting color shifting, chromaticity can also be used to make sure that replacement units in artworks produce the same color as an original. We can even set chromaticity thresholds for when color-shifting units should be replicated. Research on the applications for chromaticity measurements is limited, so stay tuned!
Do it yourself!
Power tubes and allow unit to warm up for one minute
Connect Asensetek Passport to mobile device
Subtract background
Establish contact between the unit and the Passport
Acquire measurements using mobile device interface
Compare experimental spectra to reference spectra
Document chromaticity measurements throughout a unit’s lifetime
Passport sensor makes contact to acquire measurements
Recommendations for Acquisition and Collections Management
•Ask the fabricator to include a sample unit as a reference at acquisition
•Turn it on!
•Document the color of the tube and the color of the gas near the electrodes
•Document the components: tracing of the glass unit, materials, transformer
•Document any condition issues/unexpected visuals
•Call your local neon fabricator
•Email me: taylorihealy@gmail.com